Remote Radiation Detection by Electromagnetic Air Breakdown
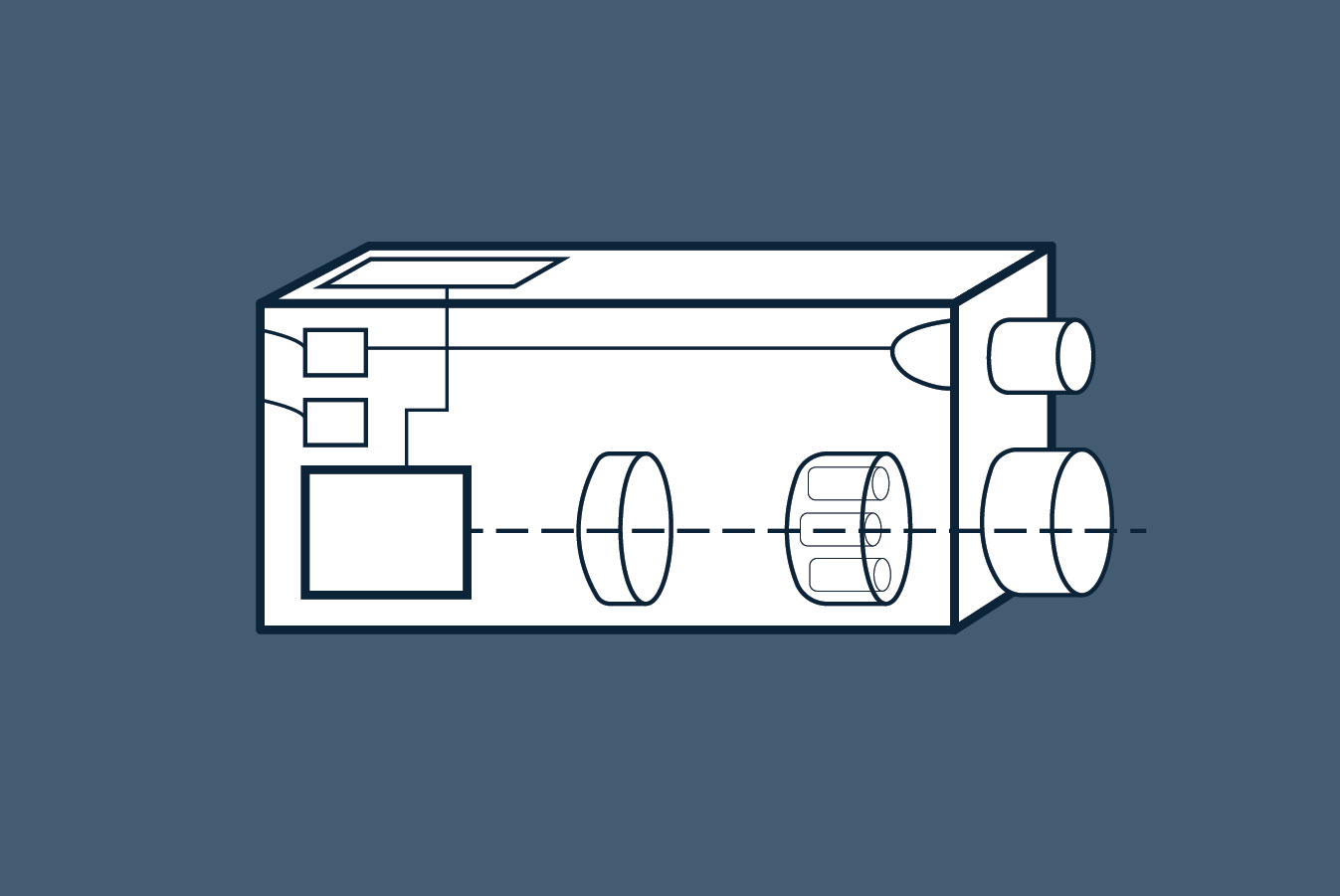
Introduction
Emerging radiation sensing techniques that measure changes in the dielectric properties of air may offer ultra-long-range radiation detection capabilities with potential applications in counterforce targeting and counterproliferation. These radiation detection systems use the reflection of high-intensity radiofrequency (RF) or infrared (IR) pulses to probe the concentration of charged species produced by radiation-induced ionization in air.1 The measurement technique is purely optical, and therefore requires no detection hardware near the radiation source. Although this family of techniques is in its infancy, lab-scale systems have been shown to be sensitive to radiation fields similar to what one would find in the immediate vicinity of a nuclear warhead or near a UF6 storage cylinder.2 With sufficient development, this technology could be used to hunt for nuclear warheads on dual-capable systems, determine the location of uranium handling facilities, and find illicit reprocessing facilities without the need for detectors deployed on the ground.
Theory of Operation
The standoff radiation-sensing platform relies on the detection of byproducts produced when ionizing radiation deposits energy in the air surrounding the source. While the details of each approach differ, the general concept is to use a source of radio waves (RF) or infrared photons (IR) to excite one of the radical species produced in air by ionizing radiation. This excitation produces a population of free electrons that can be used to seed an avalanche breakdown process. This avalanche process is created by an intense electromagnetic field generated by a secondary RF or laser sourcethat generates a small volume of plasma, the density of which evolves to reach a steady state in under a nanosecond. The initial electron population can be inferred by monitoring the time-evolution of the reflected power from the plasma. This value is directly proportional to the density of the radical species generated by the ionizing radiation and therefore to the flux of ionizing radiation in the volume. 3
Calculations performed by Kim et. al show that this method is limited by atmospheric turbulence, which diffuses the beam used to induce dielectric breakdown. Assuming a one-meter dish transmitting 30kW of 95GHz RF to induce breakdown, the authors predict a standoff distance of up 1km. However, this value may be a massive underestimate, as the authors found that their method was approximately 100 times as sensitive as predicted by simulation.4
Note: Dose-rate on contact with a DUF6 tank assumed to be ~5mR/hr (based on measurements taken on a DUF6 –filled 12B cylinder at ORNL using a H3D Polaris detector, unpublished data).
-
Joshua Isaacs, Chenlong Miao, and Phillip Sprangle, “Remote monostatic detection of radioactive material by laser-induced breakdown,” Physics of plasmas 23, no. 3 (2016): 033507. ↩
-
Dongsung Kim, Dongho Yu, Ashwini Sawant, Mun Seok Choe, Ingeun Lee, Sung Gug Kim, and EunMi Choi, “Remote detection of radioactive material using high-power pulsed electromagnetic radiation.” Nature communications 8 (2017): 15394. ↩
-
Yurii P. Raizer, “Reviews of Topical Problems: Breakdown and Heating of Gases Under the Influence of a Laser Beam,” Soviet Physics Uspekhi 8 (1966): 650–673; Alexander D. McDonald, “Microwave breakdown in gases.” (1966); Robert M. Schwartz, Daniel Woodbury, Joshua Isaacs, Phillip Sprangle, and Howard M. Milchberg, “Remote detection of radioactive material using mid-IR laser–driven electron avalanche,” Science Advances 5, no. 3 (2019). ↩
-
Dongsun Kim, et al. ↩